
( 1996), using photopigment transmittance imaging of excised patches of peripheral primate retina, reported a tendency for L and M cones to clump more than would be expected from random packing. ( 2000) showed a nasal-temporal asymmetry in L/M cone ratio in the macaque. ( 2003) both found that L:M cone ratio increases greatly in the far periphery in human retina. However, there is accumulating evidence for nonrandom arrangement of L and M cones. ( 2001) also reported a random arrangement of human foveal L/M cones for two subjects. ( 2003) reported a random arrangement of L and M cones in patches of human retina, consistent with a random assignment of these pigments to cones ( Nathans, 1999). Using microspectrophotometry, Mollon and Bowmaker ( 1992) observed a random arrangement of L and M cones in patches of talapoin monkey retina, and Bowmaker et al.
/images/6d1fc0a8/c225/48f0/ac90/e5b57cdfbd4a.jpg)
We have improved this method, expanding the number of eyes studied to more thoroughly characterize the foveal cone mosaic.Īlthough interobserver variability in L:M cone ratio is now well established, questions remain about how these cone classes are arranged within the mosaic. Roorda and Williams ( 1999) developed an in vivo method combining high-resolution retinal imaging with retinal densitometry, allowing the first direct measurements of L- and M-cone arrangement and relative numbers in the living human eye. Microspectrophotometry is not subject to this concern ( Dartnall et al., 1983 Bowmaker et al., 2003), although it is restricted to use on postmortem tissue. A limitation of these estimates is that they often involve the untested assumption that each M cone and each L cone contribute equally to the molecular, physiological, or psychophysical mechanisms being monitored to derive L:M ratio estimates. Several studies using indirect measurements have suggested that there are on average more L cones than M cones, with large intersubject variability ( DeVries, 1946 Rushton and Baker, 1964 Cicerone and Nerger, 1989 Pokorny et al., 1991 Yamaguchi et al., 1997 Hagstrom et al., 1998 Brainard et al., 1999 Deeb et al., 2000 Kremers et al., 2000 Otake and Cicerone, 2000 Carroll et al., 2002). Whereas the short-wavelength-sensitive- (S) cone submosaic has been relatively well characterized ( Williams et al., 1981 de Monasterio et al., 1985 Ahnelt et al., 1987 Curcio et al., 1991), the organization of long-wavelength-sensitive (L) and middle-wavelength-sensitive (M) cones in the human retina has proven more elusive, because they exhibit no known morphological or histochemical differences and their pigments are 96% identical ( Nathans et al., 1986). The precise topography of the trichromatic cone mosaic constrains the postreceptoral circuits that subserve spatial and color vision. Surprisingly, in the protan carrier, in which X-chromosome inactivation would favor L- or M-cone clumping, there was no evidence of clumping, perhaps as a result of cone migration during foveal development. These results suggest that the assignment of L and M pigment, although highly irregular, is not a completely random process. In a third retina, the L:M cone ratio differed significantly at two retinal locations on opposite sides of the fovea. In two retinas, these departures corresponded to local clumping of cones of like type. Although all subjects had highly disordered arrangements of L and M cones, three subjects showed evidence for departures from a strictly random rule for assigning the L and M cone photopigments. L:M cone ratio estimates were correlated highly with those obtained in the same eyes using the flicker photometric electroretinogram (ERG), although the comparison indicates that the signal from each M cone makes a larger contribution to the ERG than each L cone. All subjects had nearly identical S-cone densities, indicating independence of the developmental mechanism that governs the relative numerosity of L/M and S cones. We also found a protan carrier with an even more extreme L:M ratio (0.37:1).

As suggested by previous studies, we found males with normal color vision that varied in the ratio of L to M cones (from 1.1:1 to 16.5:1).
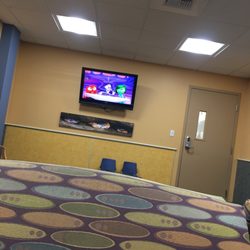
Using high-resolution adaptive-optics imaging combined with retinal densitometry, we characterized the arrangement of short- (S), middle- (M), and long- (L) wavelength-sensitive cones in eight human foveal mosaics.
